Research Experience and Mentoring (REM)
2025-2026 Undergraduate Research Experience Mentoring (REM) Program
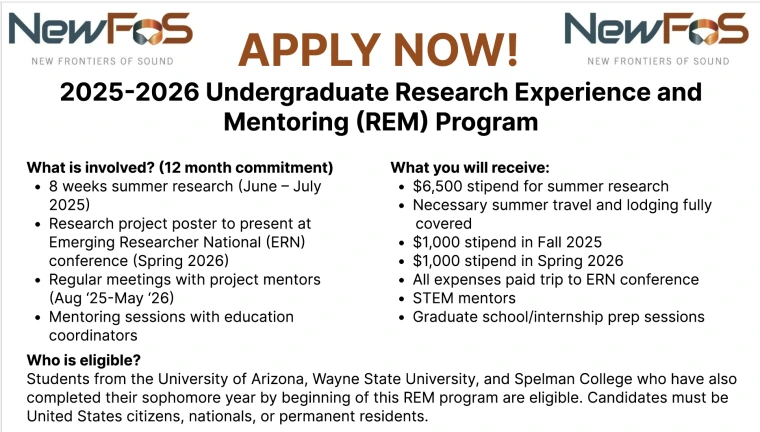
REM Project 1: WAVES: Building Emerging Technologies with Surface Acoustic Waves
Location: University of Arizona
Principal Investigator: Zafer Mutlu
Are you ready to embark on an exciting summer journey to design, build, test, and advance micro-scale Surface Acoustic Wave (SAW) devices? SAW devices utilize sound waves traveling along the surfaces of piezoelectric materials and are critical to advancements in mobile communications, medical diagnostics, environmental monitoring, and information processing. Join the Advanced Nanoelectronics & Nanostructures Group and get hands-on experience in our state-of-the-art cleanroom! You’ll learn advanced semiconductor and microfabrication techniques such as maskless lithography, deposition, and etching while working with high-tech tools to analyze and optimize device performance. No prior experience? No problem! We welcome curious and motivated students from all backgrounds in our inclusive and supportive environment. By joining this project, you’ll develop valuable skills that prepare you for exciting careers in semiconductors, radiofrequency (RF) technologies, and beyond. Are you ready to make WAVES? Let’s make it happen!
Apply today, click here!
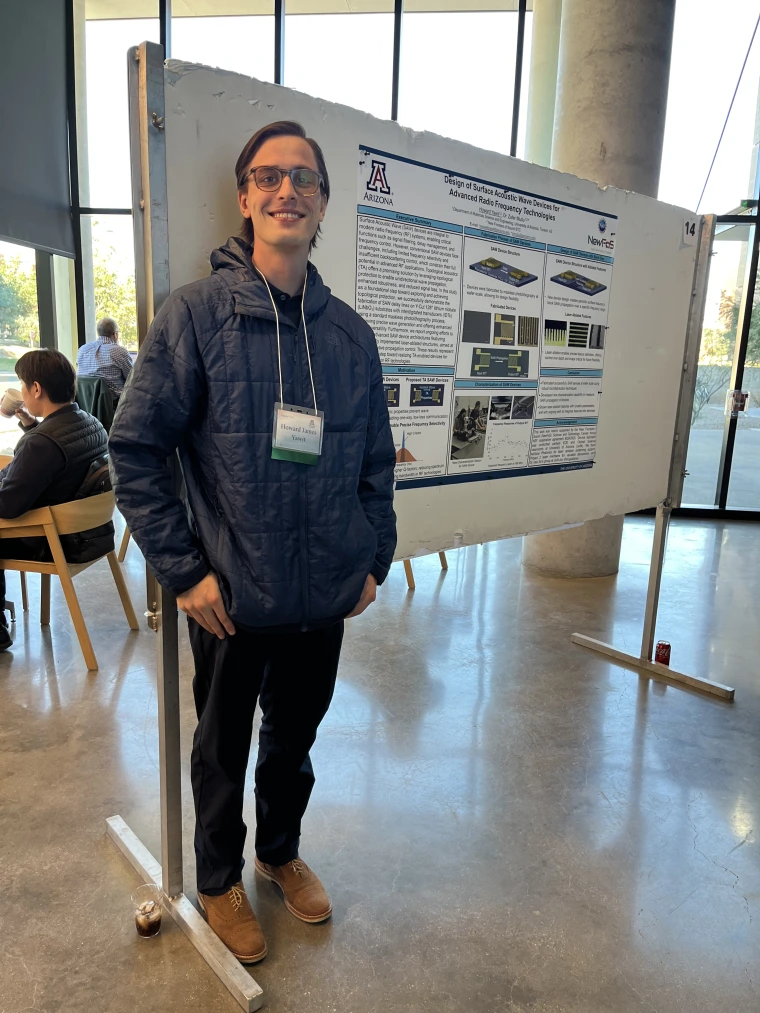
REM Project 2: Fabrication of reconfigurable acoustic devices
Location: University of Arizona
Principal Investigator: Pierre Lucas
This REM project involves the fabrication of acoustic device using phase-change materials. The phase of the material can be switched between a crystal and a glass phase, which have different elasticities. This approach can be used to build circuits that can guide acoustic waves. The project will involve two steps: first the synthesis of the phase change material from scratch, and second, the fabrication of acoustic circuits by laser writing. The REM student will therefore be trained in the use of ball mills, glove boxes and solvents for the synthesis of the phase change material. The REM student will also be trained in the use of lasers to fabricate the acoustic circuit. Finally, the fabricated waveguides will be tested in collaboration with other team from the NewFos program.
Apply today, click here!
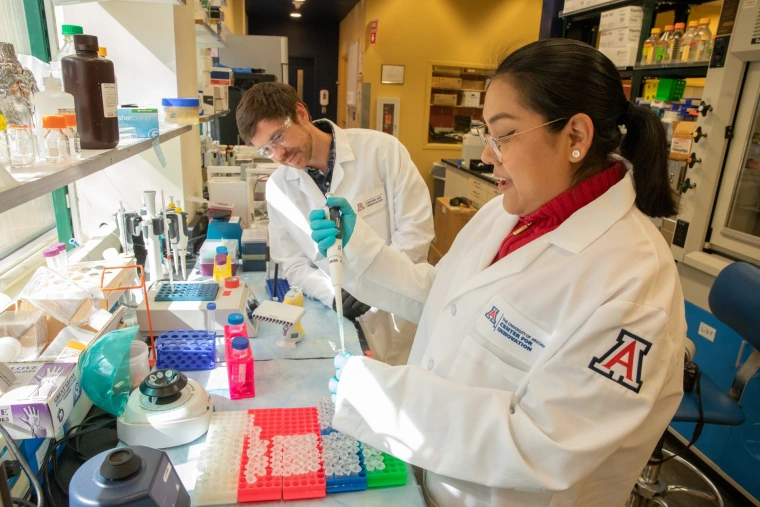
REM Project 3: Miniaturized Acoustic Waveguides for Quantum-Inspired Computing: A Finite Element Analysis Approach
Location: Wayne State University
Principal Investigator: Arif Hasan
This project proposes a rigorous exploration of miniaturized acoustic waveguides through finite element analysis (FEA), targeting advancements in quantum-inspired computing. Utilizing COMSOL Multiphysics, we will focus on the modal characteristics of single and coupled waveguide systems miniaturized via laser writing in phase change materials. We aim to model these systems' spectral, spatial, and phase characteristics to emulate quantum computing phenomena classically. These components are fundamental in harnessing and demonstrating the capabilities of phi-bits—nonlinear acoustic analogues to quantum bits (qubits). Modeling and Analysis: The core of this project revolves around the application of FEA for the detailed simulation of miniaturized coupled acoustic waveguides. Our previous work with finite-length, rod-like waveguides using COMSOL has laid a solid foundation for this endeavor. The approach will extend to more complex geometries and miniaturization levels, emphasizing the convergence of eigen- frequencies and the classification of vibrational modes essential for precise control and manipulation of phi-bits. By comparing these models to experimental results, particularly looking at longitudinal modes, we aim to refine our understanding of wave propagation in miniaturized environments. Role of the REM Students: Students will be integral to this research, contributing to the design and simulation phases. They will utilize FEA to model three-dimensional wave equations and validate their results against experimental data. They will explore nonlinear behaviors in a miniaturized system, employing advanced grid configurations to ensure simulation accuracy. Through these activities, they will gain hands-on experience in advanced computational modeling and the practical challenges of quantum computing technologies. Expected Impact: By miniaturizing coupled acoustic waveguides and analyzing them through FEA, this project not only enhances the understanding of acoustic metamaterials but also contributes significantly to the field of quantum-inspired computing. The integration of experimental and simulation approaches will help develop more accurate models for these miniaturized complex systems, potentially leading to breakthroughs in quantum analogue computing using miniaturized classical systems.
Apply today, click here!
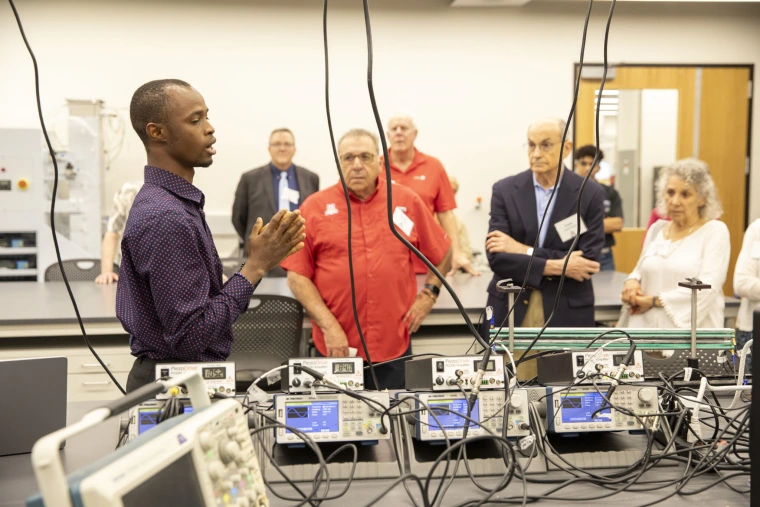
REM Project 4: Experimental Demonstration of Topological Modes in a Su–Schrieffer–Heeger (SSH) System
Location: Spelman College; Georgia Tech
Principal Investigators: Michael J. Leamy and Derrick J. Hylton
The Su–Schrieffer–Heeger (SSH) system is a simple and elegant model that exhibits topological states of matter. In its acoustics form, it describes a one-dimensional chain of masses and springs where the masses are all equal and the springs alternate periodically between soft and hard spring constants. Thus a single unit cell consisting of two equal masses, a soft spring, and a hard spring, repeats indefinitely in either direction. If this system is truncated to form two boundary-supported ends, topologically-protected edge modes can result depending on the end terminations. These edge states are robust – i.e., they persist even if the system is slightly disturbed as long as the overall pattern isn’t broken. What makes the SSH model fascinating is that it demonstrates the concept of topological protection. The edge states arise from a property of the system termed its “topological invariant,” which is commonly an integer characterizing the structure of the chain. This number changes only when the pattern (topology) of the chain changes drastically, such as when the hard and soft springs are exchanged. The SSH model is a foundation for understanding more complex systems, such as topological insulators, which have practical applications in robust electronics, guiding and channeling of acoustic energy, and quantum computing. During the summer REM program, the goal will be to experimentally demonstrate an acoustic SSH system using masses and springs supported by an air track, and to subsequently explore the robustness of the edge states to small disturbances. The experimental apparatus will be based on existing air track designs and hardware, but will be modified with the express purpose of realizing the SSH system. Students with skills in designing and fabricating mechanical designs are highly desired. Expertise with Computer-Aided Design (CAD) and 3D-printing is also desired. Students may have backgrounds in any field of engineering, mathematics, or physics. The research is expected to continue beyond the summer, with an expectation to publish results from the experiment in a conference proceedings or archival journal.
Apply today, click here!
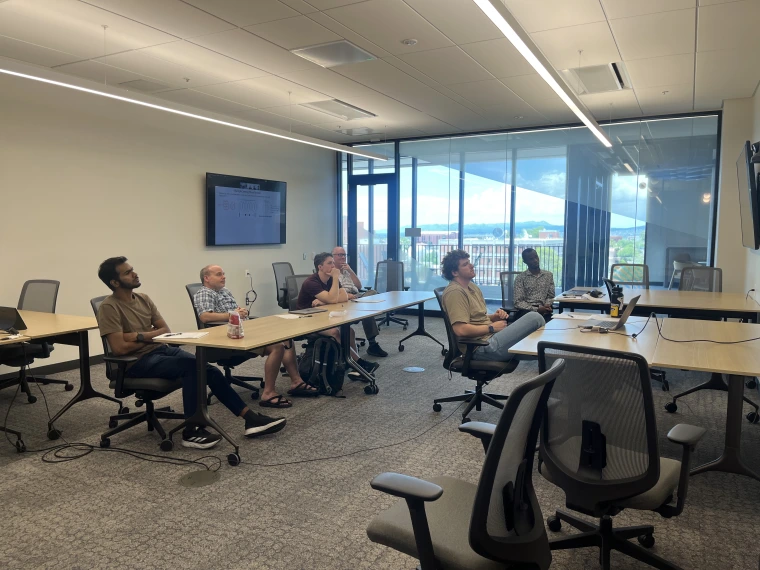